Calcium channel blockers (CCBs) are a class of medications commonly used to treat conditions such as high blood pressure (hypertension),angina (chest pain), and certain heart rhythm disorders. They work by blocking the entry of calcium into muscle cells of the heart and blood vessels, which leads to relaxation of the muscles and dilation of the blood vessels, thereby reducing blood pressure and improving blood flow to the heart.
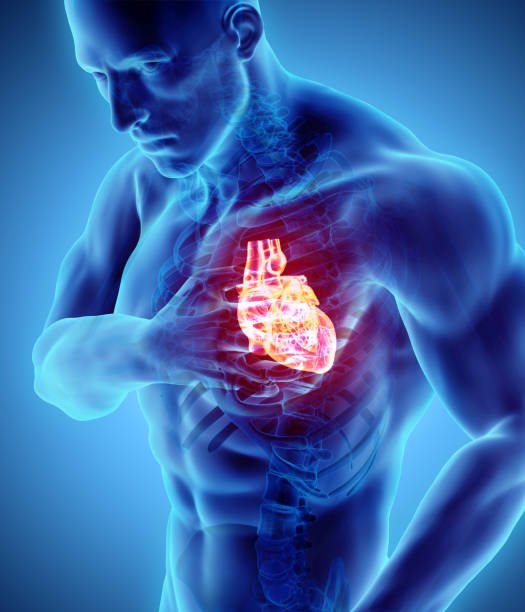
These calcium channel blockers are classified based on their chemical structure and pharmacological effects:
Dihydropyridine CCBs: This subclass primarily affects vascular smooth muscle and is commonly used to treat hypertension. They have less of an effect on cardiac tissue.
Non-Dihydropyridine CCBs: These drugs have more pronounced effects on cardiac muscle in addition to their vasodilatory effects. They are used to treat problems like angina, hypertension, and certain arrhythmias.
Here are examples of calcium channel blocker drugs along with their classification:
Dihydropyridine Calcium Channel Blockers:
- Amlodipine
- Nifedipine
- Felodipine
- Isradipine
- Nicardipine
Non-Dihydropyridine Calcium Channel Blockers:
- Diltiazem
- Verapamil
Role of calcium ions in muscles contraction and relaxation:
Calcium ions play a crucial role in muscle contraction by regulating the interaction between actin and myosin filaments within muscle fibers. Here’s a brief overview of the role of calcium ions in muscle contraction:
Initiation of Contraction: When a nerve impulse stimulates a muscle fiber, calcium ions are released from the sarcoplasmic reticulum, a specialized organelle within the muscle cell.
Troponin-Tropomyosin Complex: Calcium ions bind to troponin, a protein complex associated with the actin filaments of the muscle fiber. This binding causes a conformational change in the troponin-tropomyosin complex, exposing binding sites on the actin filaments.
Cross-Bridge Formation: With the binding sites on actin exposed, myosin heads (cross-bridges) can bind to actin, forming cross-bridges between the actin and myosin filaments.
Power Stroke: ATP is hydrolyzed to ADP and inorganic phosphate (Pi) by the myosin heads, causing a conformational change in the myosin heads. This change pulls the actin filaments toward the center of the sarcomere, resulting in muscle contraction.
Relaxation: Upon the cessation of the nerve impulse, calcium ions are actively pumped back into the sarcoplasmic reticulum, lowering the calcium ion concentration in the cytoplasm. As calcium ions dissociate from troponin, the troponin-tropomyosin complex returns to its initial shape, blocking the binding sites on actin. This prevents the calcium ions further cross-bridge formation, and the muscle relaxes.
Mechanism Of Action of Calcium Channel Blockers:
Calcium channel blockers (CCBs) work by blocking the influx of calcium ions into cells, primarily in cardiac and smooth muscle cells.
Here’s a brief overview of their mechanism of action:
Blockade of Calcium Channels: CCBs selectively inhibit voltage-gated calcium channels in the cell membrane. These channels are responsible for allowing calcium ions to enter the cell in response to membrane depolarization.
Vasodilation: In vascular smooth muscle cells, calcium influx through these channels triggers muscle contraction. By blocking calcium channels, CCBs reduce intracellular calcium levels, leading to relaxation of vascular smooth muscle and vasodilation. This dilation results in the widening of blood vessels, which reduces peripheral vascular resistance and lowers blood pressure.
Decreased Cardiac Contractility: In cardiac muscle cells, calcium influx through calcium channels is essential for initiating muscle contraction. By inhibiting calcium influx, CCBs reduce the availability of calcium ions for the contractile process. This leads to decreased myocardial contractility (negative inotropic effect), resulting in a reduction in the force of cardiac contraction.
Reduced Heart Rate: Certain CCBs, particularly non-dihydropyridine agents like diltiazem and verapamil, also affect the electrical conduction system of the heart. They can decrease the rate of spontaneous depolarization in the sinoatrial (SA) node and slow the conduction through the atrioventricular (AV) node. As a result, heart rate may be decreased, particularly in patients with certain arrhythmias or angina.
ADME Process:
Absorption:
The absorption of calcium channel blocker (CCB) drugs varies depending on their formulation and specific pharmacokinetic properties. Here’s a general overview of how CCBs are absorbed:
Oral Administration: Most CCB medications are administered orally in the form of tablets or capsules. Upon ingestion, the drugs pass through the gastrointestinal tract.
Dihydropyridine CCBs: Dihydropyridine CCBs, such as amlodipine and nifedipine, are lipophilic compounds. They are absorbed primarily in the small intestine through passive diffusion. Their absorption can be influenced by factors such as food intake, pH of the gastrointestinal tract, and drug formulations (e.g., extended-release formulations).
Non-Dihydropyridine CCBs: Non-dihydropyridine CCBs, including diltiazem and verapamil, have varying degrees of water solubility. They are absorbed in the gastrointestinal tract through passive diffusion and active transport mechanisms. Food intake may affect the absorption of some non-dihydropyridine CCBs, particularly formulations designed for sustained release.
Gastric pH and Food Intake: Gastric pH can influence the absorption of certain CCB formulations. For example, acidic environments may enhance the absorption of some CCBs, while alkaline environments may decrease absorption. Additionally, food intake can affect the rate and extent of absorption for certain CCBs. Some formulations may be recommended to be taken with food to enhance bioavailability or reduce gastrointestinal side effects.
First-Pass Metabolism: After absorption, CCBs undergo first-pass metabolism in the liver. This process may result in the formation of metabolites with varying pharmacological activity. Metabolism can also contribute to differences in the bioavailability and duration of action among different CCBs.
Plasma Concentration: Following absorption and metabolism, CCBs reach systemic circulation and exert their pharmacological effects. Plasma concentrations of CCBs can be affected by factors such as drug interactions, hepatic function, and renal function.
Distribution:
The distribution of calcium channel blocker (CCB) drugs involves their transport from the bloodstream to various tissues and organs throughout the body. Here’s an overview of the distribution of CCBs:
Plasma Protein Binding: After absorption and systemic circulation, CCBs can bind to plasma proteins, particularly albumin. This binding affects the distribution of CCBs in the bloodstream and influences their pharmacokinetics, including their volume of distribution.
Tissue Distribution: CCBs distribute to various tissues and organs in the body, including the heart, blood vessels, liver, and kidneys. The distribution of CCBs is influenced by factors such as tissue perfusion, lipid solubility, and the presence of specific drug transporters.
Cardiac Effects: CCBs exert their therapeutic effects on the heart by binding to calcium channels in cardiac muscle cells. Dihydropyridine CCBs, such as amlodipine and nifedipine, primarily affect vascular smooth muscle but may also have some cardiac effects. Non-dihydropyridine CCBs, such as diltiazem and verapamil, have more pronounced effects on cardiac muscle and can affect heart rate, contractility, and conduction.
Vascular Effects: CCBs distribute to blood vessel walls, where they exert their vasodilatory effects by blocking calcium channels in vascular smooth muscle cells. This vasodilation results in relaxation of blood vessels, reduction of peripheral vascular resistance, and lowering of blood pressure.
Hepatic and Renal Effects: CCBs are metabolized in the liver and excreted via the kidneys. Some CCBs, such as verapamil, undergo extensive hepatic metabolism, which may affect their distribution and elimination. Renal impairment can alter the pharmacokinetics of CCBs, leading to changes in their distribution and elimination.
Blood-Brain Barrier (BBB): Most CCBs are lipophilic compounds that can penetrate the blood-brain barrier to some extent. However, their distribution to the central nervous system is generally limited compared to their distribution to peripheral tissues.
Metabolism:
The metabolism of calcium channel blocker (CCB) drugs involves the biotransformation of these medications into metabolites that may have different pharmacological activities or are rendered inactive for elimination from the body. Here’s an overview of the metabolism of CCBs:
Hepatic Metabolism: Most CCBs undergo extensive metabolism in the liver, primarily through hepatic cytochrome P450 (CYP) enzymes. The specific CYP enzymes involved vary depending on the CCB drug.
Dihydropyridine CCBs: Dihydropyridine CCBs, such as amlodipine, nifedipine, and felodipine, are metabolized primarily by the CYP3A4 enzyme system in the liver. Some dihydropyridine CCBs may also undergo metabolism by other CYP enzymes, such as CYP2C9 and CYP2D6.
Non-Dihydropyridine CCBs: Non-dihydropyridine CCBs, including diltiazem and verapamil, are metabolized by multiple CYP enzymes, including CYP3A4, CYP2C9, and CYP2D6. These drugs may also undergo metabolism by other pathways, such as glucuronidation.
Metabolites: The metabolism of CCBs can result in the formation of active or inactive metabolites. In some cases, metabolites may contribute to the pharmacological effects of the parent drug. For example, diltiazem and verapamil undergo metabolism to several active metabolites, some of which retain calcium channel blocking activity.
Drug Interactions: Because CCBs are substrates for hepatic CYP enzymes, they have the potential to interact with other drugs that affect these enzymes. Concomitant use of CCBs with CYP3A4 inhibitors (e.g., azole antifungals, macrolide antibiotics) can inhibit the metabolism of CCBs, leading to increased plasma concentrations and potential toxicity. Conversely, CYP3A4 inducers (e.g., rifampin, St. John’s wort) may decrease CCB plasma concentrations, reducing their efficacy.
Genetic Variability: Genetic polymorphisms in CYP enzymes can influence the metabolism of CCBs and contribute to interindividual variability in drug response and susceptibility to adverse effects.
Renal Elimination: While hepatic metabolism is the primary route of elimination for most CCBs, some metabolites may undergo further metabolism in the kidneys before excretion in the urine.
Excretion:
The excretion of calcium channel blocker (CCB) drugs involves the elimination of these medications and their metabolites from the body, primarily through renal and hepatic pathways. Here’s an overview of the excretion of CCBs:
Renal Excretion: The kidneys play a significant role in the elimination of CCBs and their metabolites from the body. After metabolism in the liver, CCBs and their metabolites are excreted into the urine. Renal excretion is particularly important for hydrophilic CCBs and their water-soluble metabolites.
Urinary Elimination: CCBs and their metabolites are filtered from the bloodstream into the renal tubules by the glomeruli. They can then undergo reabsorption or remain in the tubular lumen for excretion in the urine. Renal elimination contributes to the clearance of CCBs from the body and helps maintain steady-state plasma concentrations.
Hepatic Elimination: While renal excretion is the primary route of elimination for most CCBs, hepatic elimination also plays a role, particularly for metabolites that undergo enterohepatic circulation. Some CCB metabolites may be excreted into the bile and eliminated in feces.
Biliary Excretion: Biliary excretion is another pathway through which CCBs and their metabolites can be eliminated from the body. CCBs that are excreted into the bile may undergo reabsorption in the intestines or be eliminated in feces.
Enterohepatic Circulation: Some CCBs and their metabolites may undergo enterohepatic circulation, where they are reabsorbed from the intestines into the bloodstream after being excreted in bile. This process can prolong the elimination half-life of CCBs and contribute to their pharmacokinetic profile.
Factors Affecting Excretion: Various factors can influence the excretion of CCBs, including renal function, hepatic function, drug interactions, and patient characteristics. Renal impairment can reduce the clearance of CCBs, leading to prolonged elimination half-lives and increased risk of drug accumulation. Similarly, hepatic impairment can affect the metabolism and elimination of CCBs, particularly for drugs that undergo extensive hepatic metabolism.
Over dosage of calcium channel blocker drugs:
An overdose of calcium channel blockers (CCBs) can lead to serious and potentially life-threatening complications due to their effects on the cardiovascular system. Here are some potential consequences and management strategies for CCB overdose:
Hypotension (Low Blood Pressure):
CCB overdose can cause severe hypotension, leading to symptoms such as dizziness, weakness, fainting, and shock. Severe hypotension can result in organ damage due to reduced blood flow to vital organs.
Management: Treatment of hypotension may involve intravenous fluids to expand blood volume, vasopressors to increase blood pressure, and supportive measures such as elevating the legs and maintaining adequate oxygenation.
Bradycardia (Slow Heart Rate):
Some CCBs, particularly non-dihydropyridine agents like diltiazem and verapamil, can cause bradycardia, which can lead to inadequate blood perfusion to tissues and organs.
Management: In cases of severe bradycardia, interventions such as atropine, intravenous fluids, and cardiac pacing may be necessary to increase heart rate and improve cardiac output.
Cardiac Conduction Abnormalities:
Calcium Channel Blockers overdose can result in various cardiac conduction abnormalities, including atrioventricular (AV) block and ventricular arrhythmias, which can be life-threatening.
Management: Prompt intervention with antiarrhythmic medications, electrical cardioversion, or temporary pacing may be necessary to restore normal cardiac rhythm and prevent hemodynamic compromise.
Metabolic Acidosis:
Severe CCB overdose can lead to metabolic acidosis due to tissue hypoperfusion and impaired cellular metabolism.
Management: Treatment of metabolic acidosis may involve intravenous administration of sodium bicarbonate to correct acid-base imbalance and improve tissue perfusion.
Hyperglycemia:
CCB overdose can cause hyperglycemia by inhibiting insulin release from pancreatic beta cells and promoting glucose release from the liver.
Management: Blood glucose levels should be closely monitored, and insulin or glucose-lowering agents may be administered as needed to control hyperglycemia.
Multi-Organ Failure:
In severe cases of CCB overdose, prolonged hypotension and tissue hypoperfusion can lead to multi-organ failure, including acute kidney injury, acute respiratory distress syndrome (ARDS), and hepatic dysfunction.
Management: Supportive care in an intensive care unit (ICU) setting, including hemodynamic monitoring, renal replacement therapy, and mechanical ventilation, may be necessary to manage complications of multi-organ failure.
Contraindications calcium channel blockers:
Calcium channel blockers (CCB) have several contraindications, meaning situations where their use is not recommended or should be avoided due to potential risks. Here are some common contraindications associated with CCBs:
Hypersensitivity: Patients who have had an allergic reaction or hypersensitivity to any calcium channel blocker medication or its components should not use CCBs.
Heart Block: CCBs, particularly non-dihydropyridine agents like diltiazem and verapamil, can slow down the conduction of electrical impulses in the heart. Therefore, they are generally contraindicated in patients with certain types of heart block, such as second- or third-degree atrioventricular (AV) block, unless a functioning pacemaker is in place.
Sick Sinus Syndrome: CCBs can exacerbate sinus node dysfunction in patients with sick sinus syndrome, leading to symptomatic bradycardia or sinus arrest. They should be used with caution or avoided in these patients.
Hypotension: Calcium Channel Blockers can cause significant vasodilation, leading to a drop in blood pressure. Therefore, they are contraindicated in patients with severe hypotension or cardiogenic shock.
Severe Heart Failure: CCBs may worsen heart failure symptoms in patients with severe left ventricular dysfunction. They are generally contraindicated in patients with acute decompensated heart failure or those with systolic heart failure who are not adequately controlled on standard heart failure medications.
Severe Liver Disease: Patients with severe hepatic impairment may have reduced metabolism and clearance of CCBs, leading to increased drug concentrations and potential toxicity. CCBs should be used with caution or avoided in these patients.
Pregnancy and Breastfeeding: The safety of CCB use during pregnancy and breastfeeding varies depending on the specific medication. Some Calcium Channel Blockers may be used with caution during pregnancy if the potential benefits outweigh the risks, while others are contraindicated due to potential adverse effects on the fetus or newborn.
Drug Interactions: CCBs can interact with other medications, including certain antiarrhythmics, beta-blockers, and statins, among others. Concomitant use of Calcium Channel Blockers with these drugs may lead to additive effects on blood pressure, heart rate, or cardiac conduction, increasing the risk of adverse events.
Pingback: Is Amlodipine Good For High Blood Pressure? - Pharmacyinfos